A new way to separate proteins?

Dmitry Matyushov, professor in the School of Molecular Sciences and the Department of Physics and author of this study.
Protein separation, purification and concentration play a huge role in understanding and treating many serious diseases as well as the development of biological tools, including biosensors. Arizona State University’s Dmitry Matyushov, professor in the School of Molecular Sciences and the Department of Physics, has spent many years absorbed in a theoretical study aimed at understanding how proteins are affected by an electric field.
Proteins in our bloodstream experience either an attraction or repulsion when exposed to a gradient of an external electric field. This effect is known as dielectrophoresis.
In a single-author article recently published in Biomicrofluidics, Matyushov suggests that the mobility of proteins in the field gradient is 10,000 times greater than anyone has previously thought.
In fact, in the past, scientists have been very reluctant to localize proteins using dielectrophoresis because theoretical calculations predicted failure. In the wake of Matyushov’s recent theoretical calculations, a number of labs worldwide are finding some very encouraging results. Professors in the School of Molecular Sciences working in this area include Alexandra Ros and Mark Hayes.
“In this work, Dmitry demonstrates another way in which liquids at interfaces are remarkably different from the bulk,” said President’s Professor Ian Gould, interim director of the School of Molecular Sciences, which is part of The College of Liberal Arts and Sciences. “This work will change our understanding of how biological molecules are solvated in polar liquids and will be critical to the development of quantitative understandings of many widely used separation techniques for biomolecules and biological structures, such as electrophoresis and bio-microfluidics.”
“This research is all about the electrostatics of water interfaces and how the structure and polarization of interfacial water affects mobility in aqueous solutions,” explained Matyushov. “Proteins are poised to show 'gigantic' dielectrophoresis when compared to the standard predictions.”
Ronald Pethig, emeritus professor of bioelectronics at the University of Edinburgh in Scotland, is excited about the new possibilities: “Professor Matyushov has published many innovative and important papers within the fields of soft matter physics and physical chemistry — but of particular interest and excitement to me is his theoretical work on dielectrophoresis,” he said.
New possibilities
The dielectric constant of a substance can be thought of as its ability to insulate charges one from another. The higher the dielectric constant, the higher the polarity and the greater the ability of the substance to stabilize charges. The dielectric constant of bulk water, 78, does not at all characterize interfaces.
The polar response of water at an interface is very different from the bulk and depends on the charge of the solute and its distribution. Since water molecules are asymmetric, they adopt spontaneous polarization at the interface.
This can be seen as a non-zero electrostatic potential created by water in a void. The research described here connects this potential to an effective surface charge of interfacial water and its effective dielectric constant at the interface.
The actual magnitude of the interfacial dielectric constant is still disputed and has not been conclusively measured, but it must be much lower than in the bulk, in the range of four to 10. There are observable consequences of constrained polarization of interfacial water, ranging from mobility of small ions to dielectric spectroscopy of protein solutions and the response of proteins to electric field gradient.
This theory connects the positive potential within the void in water with the effective negative charge that voids show by their mobility when placed in an external field. It also shows why proteins in solutions are much more sensitive to the external electric field than is suggested by the standard theories of dielectrics. The latter result has direct application to technologies of separation of proteins from solutions by applying electric field gradient (electrophoresis).
“The major advantage of dielectrophoretic manipulation is that it is a label-free technique, where — under ideal conditions — particles of the same type can be selectively immobilized and then released by simply changing the frequency of the applied electric field signal,” said Pethig. “For example, commercial devices for the dielectrophoretic isolation of metastatic cancer cells from whole blood have been clinically tested, and it is hoped that the demonstration of fast capture and release of rRNA units opens up new opportunities for rRNA-based biosensing. The ability to selectively manipulate proteins based on their subtle dielectric characteristics opens up even more exciting opportunities.”
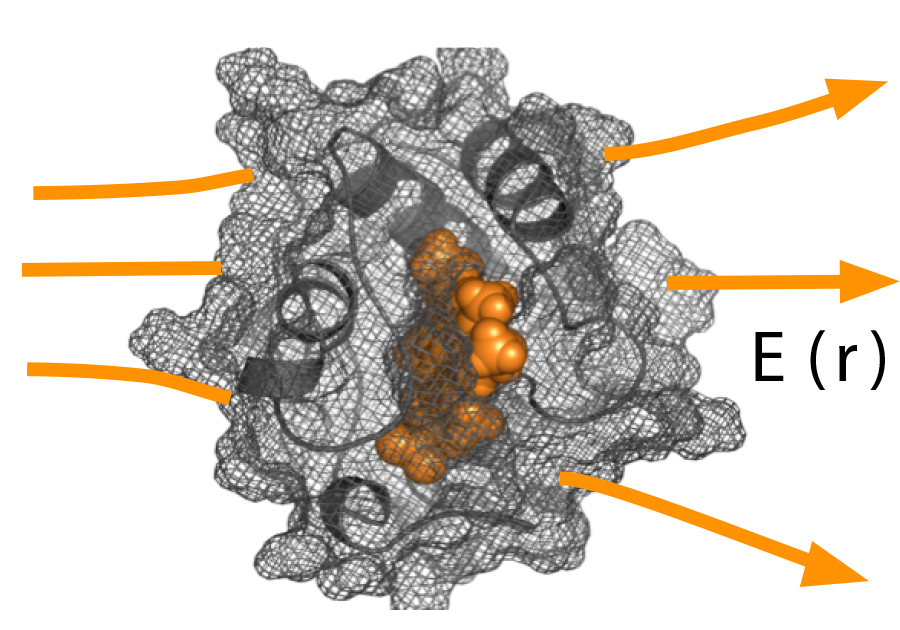
The mobility of proteins in the field gradient is 10,000 times greater than anyone has previously thought.
Applications in the real world
Proteins dissolved in water should show large dielectrophoretic susceptibility, about 10,000 greater than thought so far. This result will allow one to separate proteins from solutions by capturing them with electric field gradients.
Matyushov’s theory also explains the spontaneous charging of bubbles and oil drops in water. Gas bubbles have been known to be negatively charged in water for longer than a century. The effect had been traditionally traced to adsorption of hydroxyls to the interface, but recent direct experiments have shown little evidence of the presence of those ions at the interface. The new theory places the emphasis on the spontaneous orientation of water at the interface.
The medical field is currently experiencing huge demands on the improvement of protein analysis tools. Matyushov’s current theory is already driving an interest in protein dielectrophoresis as well as the improvement of micro- and nanofabrication techniques applied to protein dielectrophoresis — e.g., as sensing tools or in microfluidic devices.
“I believe this work heralds a new era for dielectrophoresis by providing a theoretical framework that takes the subject from the microscopic world of cells and bacteria down to the molecular scale of proteins (and hopefully also nucleic acids),” explained Pethig. “Subtle differences between proteins can in principle lead to large differences in their dielectrophoretic behavior. For example, there is no clear correlation between a protein’s size (molecular weight) and its intrinsic dipole moment. The baton has now passed to the experimentalist — who must ensure that such factors as uncontrolled electrohydrodynamic and joule heating effects in their microfluidic devices do not confuse matters when testing this beautiful theory.”
This research was supported by the National Science Foundation (NSF) (No. CHE-1800243).
More Science and technology

Podcast explores the future in a rapidly evolving world
What will it mean to be human in the future? Who owns data and who owns us? Can machines think?These are some of the questions…
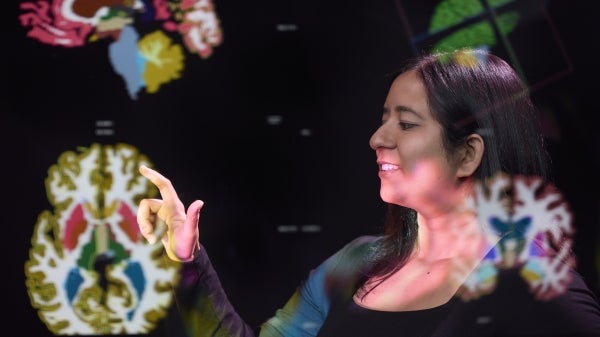
New NIH-funded program will train ASU students for the future of AI-powered medicine
The medical sector is increasingly exploring the use of artificial intelligence, or AI, to make health care more affordable and…
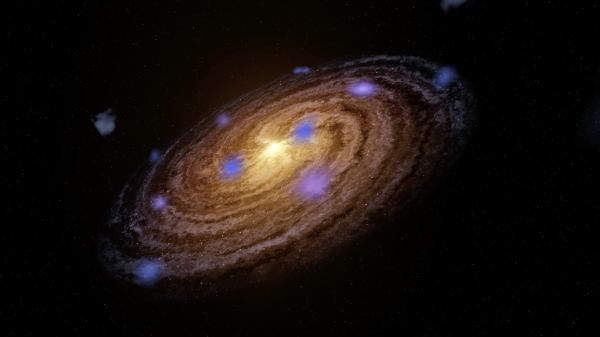
Cosmic clues: Metal-poor regions unveil potential method for galaxy growth
For decades, astronomers have analyzed data from space and ground telescopes to learn more about galaxies in the universe.…