Breathing with rocks as an energy source
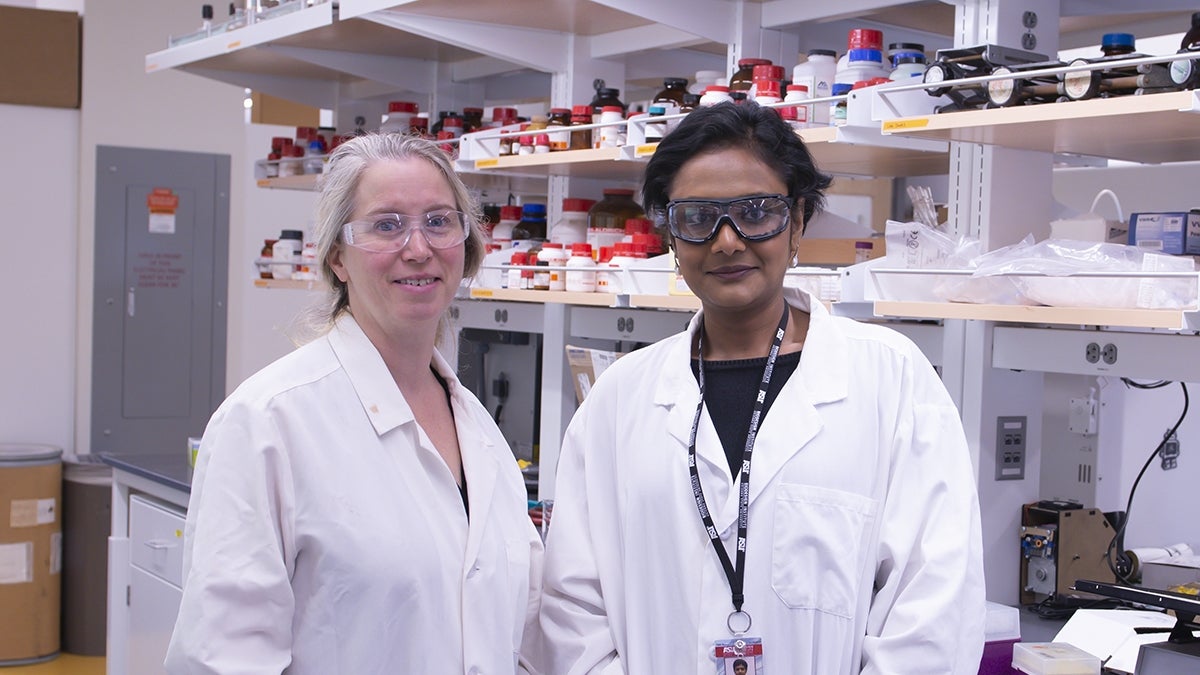
ASU School of Molecular Sciences Professor Anne Jones (left) and postdoctoral researcher Miyuki Thirumurthy.
Cells are the building blocks of life, but in order to generate life-sustaining energy, they need to breathe. They do this through a complex series of chemical reactions known as cellular respiration in which molecules are oxidized and reduced as electrons are transferred down the chain. The process ends with the electrons being trapped by molecular oxygen within the cell.
While the usual process requires oxygen, some cells are able to undergo respiration without it through a process called extra-cellular electron transfer (EET), in which they pass electrons to structures completely outside the cell, to be trapped in iron- or manganese-containing minerals instead.
In short, these cells use rocks to breathe.
The notion that electrons, and therefore energy, can be extracted from cells this way has considerable scientific interest for use in such diverse applications as fuel cells, biosensors and the electrochemical synthesis of new and useful materials.
But to build effective devices using EET, the detailed molecular mechanisms of the process need to be understood. For example, what are the molecular structures that carry the electrons across the cell membrane and beyond? These are the questions that Arizona State University postdoctoral researcher Miyuki Thirumurthy and Professor Anne Jones of the School of Molecular Sciences are answering, and their work was recently published in the journal Nanotechnology.
Cytochromes are proteins involved in many different kinds of electron transfer processes in cells. Thirumurthy and Jones have now found that a specific protein, OmcZs, can shuttle electrons to a soluble mediator molecule — a riboflavin — that is produced by the cells and is secreted to the outside world. In this way, riboflavin can transfer an electron to extracellular objects like insoluble minerals before returning to the cells to collect more electrons, repeating the process.
OmcZs is found in the organism Geobacter sulfurreducens, a bacteria that is known to undergo EET. Thirumurthy and Jones were able to express a soluble form of the protein from G. sulfurreducens in the organism E. coli, and then used it to study its molecular interactions with riboflavins.
They were able to show that riboflavin and OmcZs undergo strong binding, and that direct electron transfer from the protein to riboflavin occurs efficiently. These observations suggest a mechanism whereby the protein accepts electrons at the end of the respiration reaction chain, shuttles the electrons across the cell membrane and hands them off to a water-soluble riboflavin.
Schematic diagram showing how the multi-heme centered protein OmcZs can shuttle electrons across the outer membrane of a cell and couple to a water-soluble riboflavin molecule. The riboflavin then transfers the electrons to an electrode, effectively coupling cellular metabolic processes to an external electron sink while creating electricity that can be used to generate power or drive useful chemical reactions.
Unlike G. sulfurreducens, E. coli does not undergo EET. However, Thirumurthy and Jones also made the remarkable observation that an E. coli expressing OmcZs gained the ability to undergo EET itself. Experiments in a bioelectrochemical cell in the presence of a water-soluble riboflavin showed that an electrical current could be generated as a result of extracellular electron transfer using OmcZs from the metabolic apparatus of E. coli to an external electrode.
“Researchers have known for quite a while that OmcZs is one of the most important proteins in extracellular electron transfer by Geobacter, but this is the first study to start to show why, and to define what the protein is doing,” Jones said. “Perhaps more importantly, we also show that this protein alone is sufficient to introduce a completely new functionality into E. coli.”
One reason EET is so important is that it shows how nature solved a problem that scientists have found to be extremely challenging, which is how to connect biological and electronic electrical systems.
Thirumurthy and Jones’ work can be used to help create microbes that can readily communicate with different environments for application in self-repairing sensors, electronics and power generation.
Written by Ian Gould, School of Molecular Sciences
More Science and technology
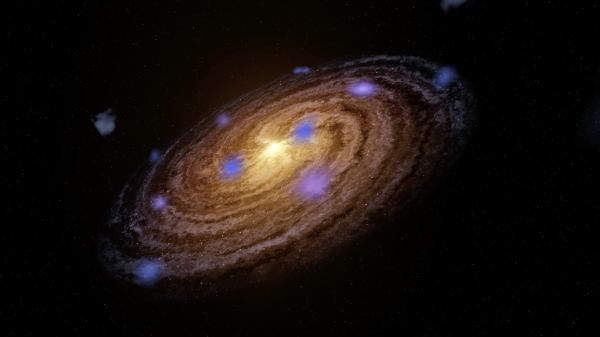
Cosmic clues: Metal-poor regions unveil potential method for galaxy growth
For decades, astronomers have analyzed data from space and ground telescopes to learn more about galaxies in the universe.…
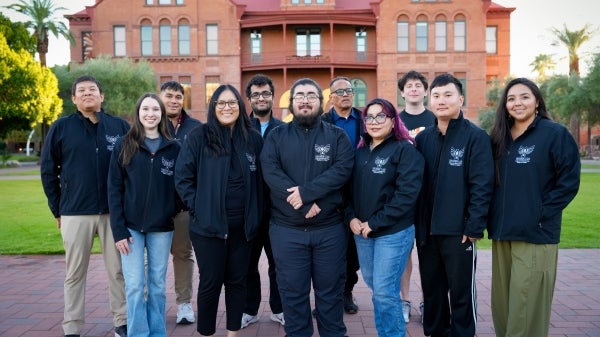
Indigenous geneticists build unprecedented research community at ASU
When Krystal Tsosie (Diné) was an undergraduate at Arizona State University, there were no Indigenous faculty she could look to…

Pioneering professor of cultural evolution pens essays for leading academic journals
When Robert Boyd wrote his 1985 book “Culture and the Evolutionary Process,” cultural evolution was not considered a true…