Researchers explores genome fidelity, its consequences for cellular health
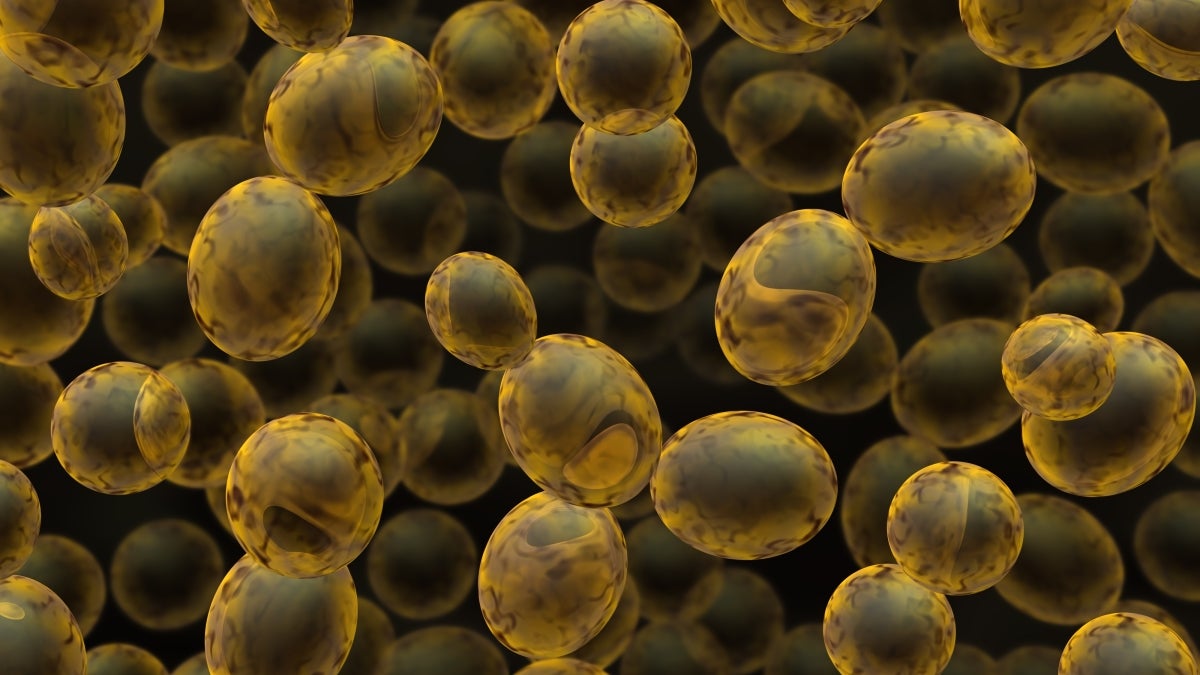
A study on how sporadic mutations affect cell function may also provide a new window into the understanding of non-inherited forms of human disease.
It’s biology’s version of the whisper game. Inside a cell, every DNA phrase or sentence that makes a protein, known as a gene, first must be precisely copied, to ensure its instructions can properly build the foundation of life.
But much like children tasked in the game with faithfully whispering a phrase to one another, each time, there is the possibility of introducing errors when the DNA information is passed along inside every living cell.
In biology, scientists have long wanted to explore this fidelity and how much random error a cell can handle before things really start to go haywire and affect its survival.
Now, in a large survey, a team of researchers, has come to grips with this issue in a study that has both implications for understanding how sporadic mutations affect cell function, but also, may provide a new window into understanding non-inherited forms of human disease.
“Our observations demonstrate that there is an inherent limit to the faithful expression of the genome, and suggest that the impact of mutagenesis on cellular health and fitness is substantially greater than currently appreciated,” said ASU Biodesign Institute researcher Michael Lynch, who was recently recruited to ASU as director of the Biodesign Center for Mechanisms of Evolution and professor in the School of Life Sciences.
If DNA is the blueprint of life, faithfully copying out its instructions inside every cell make proteins, which are the architect’s bricks and mortar of every cell. There is an intermediary in this transaction, called RNA. In a process called transcription, the DNA blueprint must first be copied into RNA, from which it can eventually make proteins.
Unlike genetic mutations, transcription errors are transient events that are not stably inherited from cell to cell, which, until now, has made them difficult to detect.
In a collaboration with Marc Vermulst at the Children’s Hospital of Philadelphia, Lynch’s research team, which included critical contributions from postdoctoral researcher Jean-Francois Gout and graduate student Weiyi Li, has developed a powerful new sequencing technology to provide the first comprehensive analysis of the fidelity of transcription across the genome with single letter resolution (of the DNA chemical bases G,C,A,T (or U in the case of RNA).
“Sporadic errors are unavoidable though, and these errors reveal how important biological fidelity is for organismal health,” said Vermulst, whose lab is focused on understanding how these errors may contribute to the mechanisms of aging.
One theory is that, much like a high-mileage car, things inside the cell start to wear or break down, including copying DNA.
“For example, errors that occur during DNA replication contribute to carcinogenesis, while errors that occur during transcription and translation induce protein aggregation, which has been implicated in a range of diseases including Alzheimer’s and Parkinson’s.”
This protein pile-up is like a multi-car accident on the freeway, clogging up normal cell functions.
“... There is an inherent limit to the faithful expression of the genome, and suggest that the impact of mutagenesis on cellular health and fitness is substantially greater than currently appreciated,” said Michael Lynch, who was recently recruited to ASU as director of the Biodesign Center for Mechanisms of Evolution and professor in the School of Life Sciences.
To determine the error rate of transcription, the research team analyzed more 2.5 billion bases from 12 biological replicates of healthy yeast cells, a model organism used by biologists because of its ease of genetic manipulation.
They found that on average the yeast transcriptome contains about 4.0 errors per million base pairs. That may not sound like much, but their results demonstrate that transcription errors occur greater than 100-fold more frequently than when DNA is copied every time a cell divides, known as DNA replication errors.
“Our experiments represent the first comprehensive analysis of the fidelity of transcription in a eukaryotic organism,” Lynch said. “The errors we detected were distributed across the entire transcriptome of S. cerevisiae, indicating that our approach provides a genome-wide view of transcriptional mutagenesis in yeast.”
Transcription errors play an important role in protein stability. For example, in humans, transcription errors generate toxic versions of the Aβ protein in patients with non-familial Alzheimer’s disease and faulty ubiquitin-B proteins in patients with Down syndrome. In addition, transcription errors induce proteotoxic stress and accelerate cellular aging in yeast.
Most likely, these observations directly underlie the link between transcription errors and misfolded proteins inside cells. The team has previously shown, and confirmed in their latest experiments, that these misfolded proteins can affect both the growth rate and lifespan of yeast cells.
To better understand the link between transcription errors and protein instability, they examined the impact of transcription errors on proteins in greater detail. The took advantage of some tricks of yeast genetics so that could tweak the genetic background, hijacking these mutant cells to increase the transcription error rate in comparison to normal cells.
Overall, they identified 21 genes that were significantly upregulated in error-prone cell lines.
A whole-proteome analysis was performed, sifting through more than 4000 proteins and demonstrating that 12 of these 21 genes were also upregulated more than 2-fold higher at the protein level in both error-prone cell lines.
Five of these genes play a role in protein quality control, consistent with the idea that transcription errors result in proteotoxic stress.
“Surprisingly though, we found that the remaining genes were involved in various metabolic pathways,” Lynch said.
“Together, these experiments provide evidence for the idea that in addition to proteotoxic stress, transcription errors can also lead to widespread changes in the metabolism of eukaryotic cells, possibly due to the depletion of vital resources,” Lynch said.
“In addition, we describe how numerous proteins maintain the fidelity of transcription.
Similar experiments could determine how age, nutrition, genotype or exposure to chemicals affects the error rate of transcription, or whether transcriptional fidelity is perturbed in the context of human disease,” Lynch said.
With the technology, the team has opened up a new and exciting avenue of research.
“It will be possible to define the transcriptional component of these non-genetic mutations for the very first time and to understand how this molecular noise affects cellular function. Together, these considerations indicate that our experiments open up a new field of mutagenesis to widespread experimentation,” Lynch said.
One of the most challenging aspects of this field will be to define the impact of transcription errors on cellular health.
“We anticipate that these experiments will ultimately lead to the discovery of a wide range of unexpected phenomena, including new mutagens, new mutational mechanisms, and new disease processes, that could help us understand how the environment and our lifestyle choices affect our overall health, as well as our predisposition to diseases that are caused by protein aggregation,” Lynch said.
More Science and technology

ASU postdoctoral researcher leads initiative to support graduate student mental health
Olivia Davis had firsthand experience with anxiety and OCD before she entered grad school. Then, during the pandemic and as a…
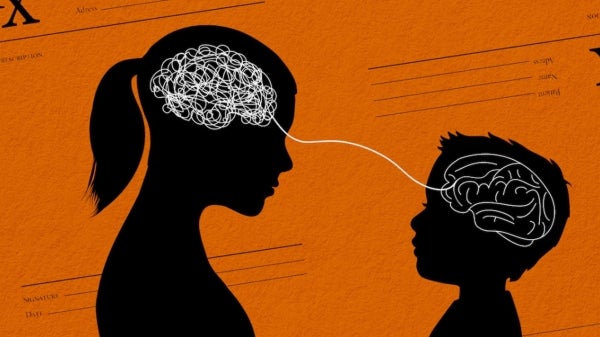
ASU graduate student researching interplay between family dynamics, ADHD
The symptoms of attention deficit hyperactivity disorder (ADHD) — which include daydreaming, making careless mistakes or taking…

Will this antibiotic work? ASU scientists develop rapid bacterial tests
Bacteria multiply at an astonishing rate, sometimes doubling in number in under four minutes. Imagine a doctor faced with a…